In August 1865, a 10-pound rock fell from space to Earth, landing with a bang in the remote village of Sherghati, India. After being recovered by witnesses to the event, the stone passed into the possession of a local British magistrate who endeavored to identify the source of the strange object. After more than a century of studying the meteorite fragments—so-called shergottites—researchers in the 1980s finally determined its alien origins: our neighboring planet, Mars.
Until humans are able to bring back samples from Mars, the only pieces of the Red Planet found on Earth are Martian meteorites such as the shergottites. The journey for these little Martian travelers has been violent: for Mars rocks to get to Earth, they must have been ejected from the Red Planet's surface with enough force to escape Martian gravity. This ejection was likely due to a large impact on Mars. The rocks withstood the massive temperatures and pressures of this impact and flew through the vacuum of space, eventually crash-landing on our own planet.
For decades, scientists have worked on modeling the kind of Martian impact events that send bits of the Red Planet to Earth. Now, researchers at Caltech and the Jet Propulsion Laboratory (JPL), which Caltech manages for NASA, have conducted experiments to simulate the so-called "shock pressure" experienced by Martian rocks. They have found that the pressure required to launch a rock from Mars into space is much lower than originally thought.
The research was conducted in the laboratory of Paul Asimow (MS '93, PhD '97), the Eleanor and John R. McMillan Professor of Geology and Geochemistry. The study is described in a paper appearing in the journal Science Advances on May 3 and is a collaboration with JPL.
Meteorites from varied sources have been discovered on Earth for millennia, but their origins were not known until much more recently. As NASA's Viking orbiters made measurements of Mars's atmospheric composition in the late 1970s, Caltech's Ed Stolper (now the Judge Shirley Hufstedler Professor of Geology) was one of the first to suggest that shergottites are from Mars—confirmed later when gases in the thin Martian atmosphere matched up with the gases encapsulated in the meteorites.
But that is not all a meteorite's composition can tell us about its journey. One major component of Martian rocks is the crystalline mineral plagioclase. Under high pressures, such as an intense impact, plagioclase transforms into the glassy material known as maskelynite. Finding maskelynite in a rock, therefore, indicates the types of pressure the sample came into contact with. In the last five years, Martian meteorites have been discovered with a blend of both plagioclase and maskelynite, indicating an upper bound for the pressures they were subjected to.
In the new study, led by Caltech staff scientist Jinping Hu, the team conducted experiments to smash plagioclase-containing rocks from Earth and observe how the mineral transforms under pressure. The team developed a more accurate method to simulate Martian impacts in shock-recovery experiments, utilizing a powerful "gun" to blast rocks with projectiles traveling over five times the speed of sound. Previous shock-pressure experiments required reverberating shock waves through a steel chamber, which gives an inaccurate picture of what happens during an impact event on Mars.
"We're not on Mars, so we can't watch a meteorite strike in person," says Yang Liu, a planetary scientist at JPL and a co-author on the study. "But we can recreate a similar kind of impact in a lab setting. By doing so, we found it takes much less pressure to launch a Mars meteorite than we thought."
Previous experiments had shown that plagioclase turns into maskelynite at a shock pressure of 30 gigapascals (GPa), which is 300,000 times the atmospheric pressure one experiences at sea level, or 1,000 times the pressure a submersible comes into contact with while diving beneath 3 kilometers of ocean water. This new study shows that the transition actually happens at around 20 GPa—a significant difference from previous experiments. In particular, the new pressure threshold is consistent with evidence from other high-pressure minerals in these meteorites indicating that their shock pressures must have been less than 30 GPa. Nine out of the 10 high-pressure minerals found in Martian meteorites were discovered at Caltech in studies led by mineralogist Chi Ma, Caltech's director of analytical facilities, and a co-author of the study.
"It has been a significant challenge to model an impact that can launch intact rocks from Mars while shocking them to 30 GPa," Asimow says. "In this context, the difference between 30 GPa and 20 GPa is significant. The more accurately we can characterize the shock pressures experienced by a meteorite, the more likely it becomes that we can identify the impact crater on Mars from which it originated."
The paper is titled "Shock-recovered maskelynite indicates low-pressure ejection of shergottites from Mars." Hu, Asimow, Liu, and Ma are co-authors. Funding was provided by NASA, Caltech-JPL, and the National Science Foundation.
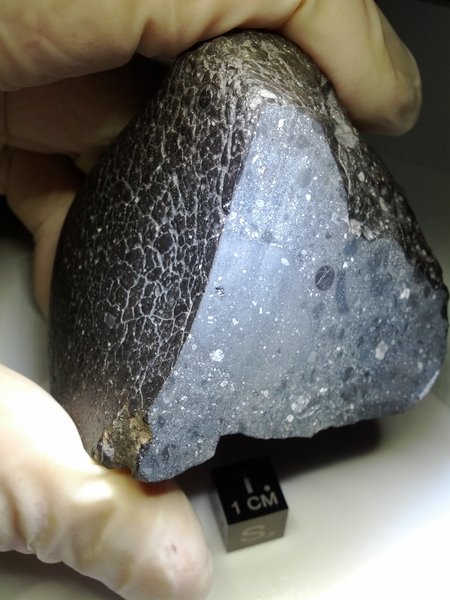
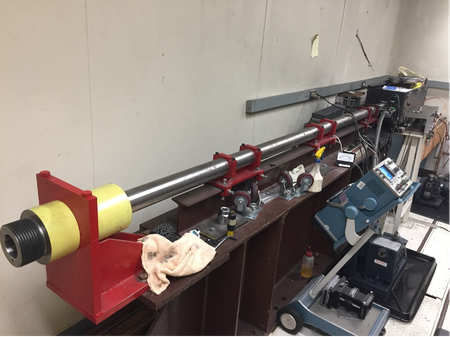
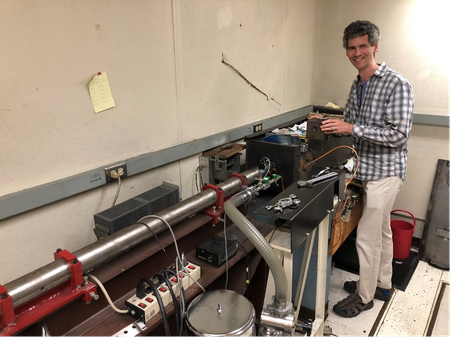
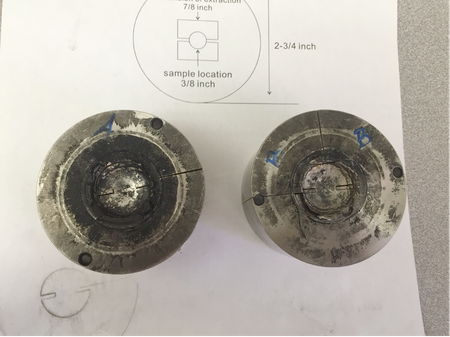