Three new faculty members in the Division of Engineering and Applied Science (EAS) have big ideas about really small things. Assistant professors Hyuck Choo, Dennis Kochmann, and Austin Minnich focus on quite different challenges, but they all home in on the nanoscale, where they manipulate, model, and measure structures and phenomena at the level of individual atoms.
"Caltech and EAS take pride in lowering the barriers between disciplines to create collaborative environments for researchers such as Hyuck, Dennis, and Austin to work on a variety of topics including understanding and predicting behavior of materials at the nanoscale, which already is an area of strength within EAS," says Ares J. Rosakis, chair of the division, Theodore von Kármán Professor of Aeronautics, and professor of mechanical engineering.
Hyuck Choo, Assistant Professor of Electrical Engineering
Electrical engineer Hyuck Choo is developing a mechanism to focus laser light down to a spot just 25 nanometers (25 billionths of a meter) in diameter. Such a tiny spotlight could help manufacturers of hard disk drives continue to increase the capacity of their disks.
A typical hard drive includes a platter where data is recorded; that platter is made up of many, many individual magnetic grains, called bits. To increase the storage capacity of these disks, developers have to keep squeezing more bits into each square inch of a platter.
But they've hit a wall of sorts. Top-of-the-line hard drive disks currently hold about 750 gigabytes (GB) per square inch. The upper limit, using current methods and materials, is 1 terabyte (1,024 GB) per square inch. However, to reach such a density, the individual bits need to be smaller than 25 nanometers, and at that size, the ferromagnetic materials they're traditionally made from become unstable at room temperature, making them unreliable for data storage.
Switching to a different material, iron-platinum alloy, for the platters, the individual bits can remain stable down to 2.5 nanometers. The only problem is that iron platinum is so stable that it has to be heated in order to record information.
That's where Choo, who is part of the Kavli Nanoscience Institute, comes in. "We are creating a laser spot that is small enough to go down to the 25-nanometer size, so that we can just heat up individual magnetic bits," Choo says. "Our solution could be used to enable the next generation of hard drive disks."
To illuminate such a miniscule spot, Choo's group has developed a device called a "plasmonic waveguide." Made of gold and glass, the three-dimensional guide channels laser light through a tapering geometry to a tip that's just 14 nanometers across. Choo explains that when they shine laser light at an angle along the waveguide, "the energy of the photons gets transferred to the electrons at the interface between the glass and the gold." Once that happens, an electron wave travels down to the tip, compressing the energy into a very small spotlight.
Choo was born in the United States, raised in South Korea, and went to high school in Worcester, Massachusetts. He did his undergrad work at Cornell University and earned his PhD at the University of California, Berkeley. In addition to the light-focusing project, Choo's lab is also working on label-free imaging for glaucoma research.
Dennis Kochmann, Assistant Professor of Aerospace
Aerospace engineer Dennis Kochmann is developing theoretical and computational models of solid materials across multiple time and length scales-from the nano all the way up to the macroscale, which we deal with on an everyday basis.
"The behaviors that you observe at the macroscale usually stem from very many mechanisms from components at lower scales," Kochmann says. "If you zoom in with a microscope on a piece of metal, which looks homogeneous to the naked eye, you will see that it has microstructure-that there are many, many very small things going on across various length scales."
For example, a metal is basically a regular lattice of atoms. But if you zoom in, that lattice is full of defects such as misplaced or completely missing atoms (so-called dislocations or vacancies). Kochmann says these innumerable defects are extremely important because they provide a material with its very distinct properties. "We need to model these lower scales in order to, on the one hand, be able to understand the behavior of materials, but also to predict the behavior of materials," Kochmann says. "If you want to design a material, you need to understand where its properties come from."
In his research, Kochmann focuses on modeling the behavior of crystalline solids bridging scales from the nano all the way up to the macro. At the lowest scale, methods of molecular dynamics help him to investigate the basic mechanisms at play within a crystal. These studies inform higher-scale engineering models and lay the basis for full-physics material models. He hopes that such complete and efficient models will help engineers simulate and predict material behavior with high accuracy.
He's also interested in designing new materials, in particular composite materials with novel properties that can be turned on and off. As a grad student, he was involved in the creation of such a material, which could be made both highly stiff and highly damping, or energy absorbent, at a particular temperature. At Caltech he plans to expand this concept to create new materials with tunable properties.
Kochmann, who was born and raised in Germany, earned his Diplom and doctoral degree in mechanical engineering from Ruhr-University Bochum. He also studied at the University of Wisconsin-Madison as a Fulbright fellow, and earned his MS in engineering mechanics there. He spent the last academic year working as a postdoctoral scholar in the lab of Michael Ortiz, the Dotty and Dick Hayman Professor of Aeronautics and Mechanical Engineering at Caltech.
Austin Minnich, Assistant Professor of Mechanical and Civil Engineering
Mechanical engineer Austin Minnich is interested in thermoelectrics-materials that are capable of converting heat directly into electricity. Thermoelectrics have been around for decades but are receiving increased attention now because they could help with energy conservation efforts. "The simplicity and reliability of thermoelectric devices offer many opportunities to recover wasted energy," Minnich says.
Thermoelectrics require an unusual combination of properties. "You're looking for high electrical conductivity and low thermal conductivity," Minnich explains. Just as electrical conduction is the flow of electrons through a material, thermal conduction refers to the flow of phonons, packets of vibrational energy, through a material. Typically, he says, a material is high in both types of conductivity or low in both. For example, metals are high in both, while glass is low in both.
But over the past decade, researchers have started to find that they can improve existing thermoelectrics by introducing nanostructures-tiny inclusions. If the inclusions are carefully sized, they can disrupt or scatter phonons, while retaining most of the material's electrical conductivity.
Minnich is focused on developing and using different tools to investigate the nanoscale physics at play in such thermoelectric materials. To properly size the nanoinclusions to scatter phonons, Minnich says it would be useful to know a measurement called the mean free path-the distance that phonons travel without being disrupted-for a variety of materials. The problem is, these values haven't been determined for most materials. "This means that we don't really know what size or distribution of sizes of nanostructures are needed to optimally scatter phonons," he says.
As part of his research program, Minnich has developed a method to measure the details of heat transport at nanometer-length scales using ultrafast lasers. Fourier's law, which governs heat transfer at the macroscopic level, no longer holds true at nanometer scales. Minnich systematically varies the size of the region heated by the laser and observes both how that heat moves through the material and how heat transfer deviates from what would be expected with Fourier's law. From those deviations, he can figure out how different phonon mean free paths contribute to the material's heat conduction.
"We use these techniques with very fine time resolution, looking at thermal conduction over periods of just femtoseconds," he says. "And that allows us to look at details of transport that are normally just lost if you look at longer time scales." So far, he's been able to measure mean free paths for phonons in silicon and sapphire, and he plans to apply the technique to many more semiconductors, including thermoelectric materials.
Minnich grew up on the East Coast and went to high school in New Mexico. He completed his undergraduate education at the University of California, Berkeley, and earned his PhD at the Massachusetts Institute of Technology.
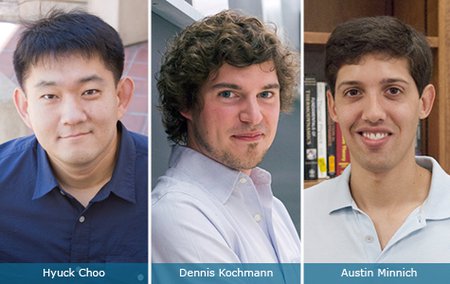
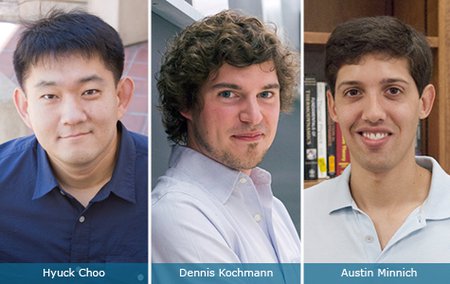